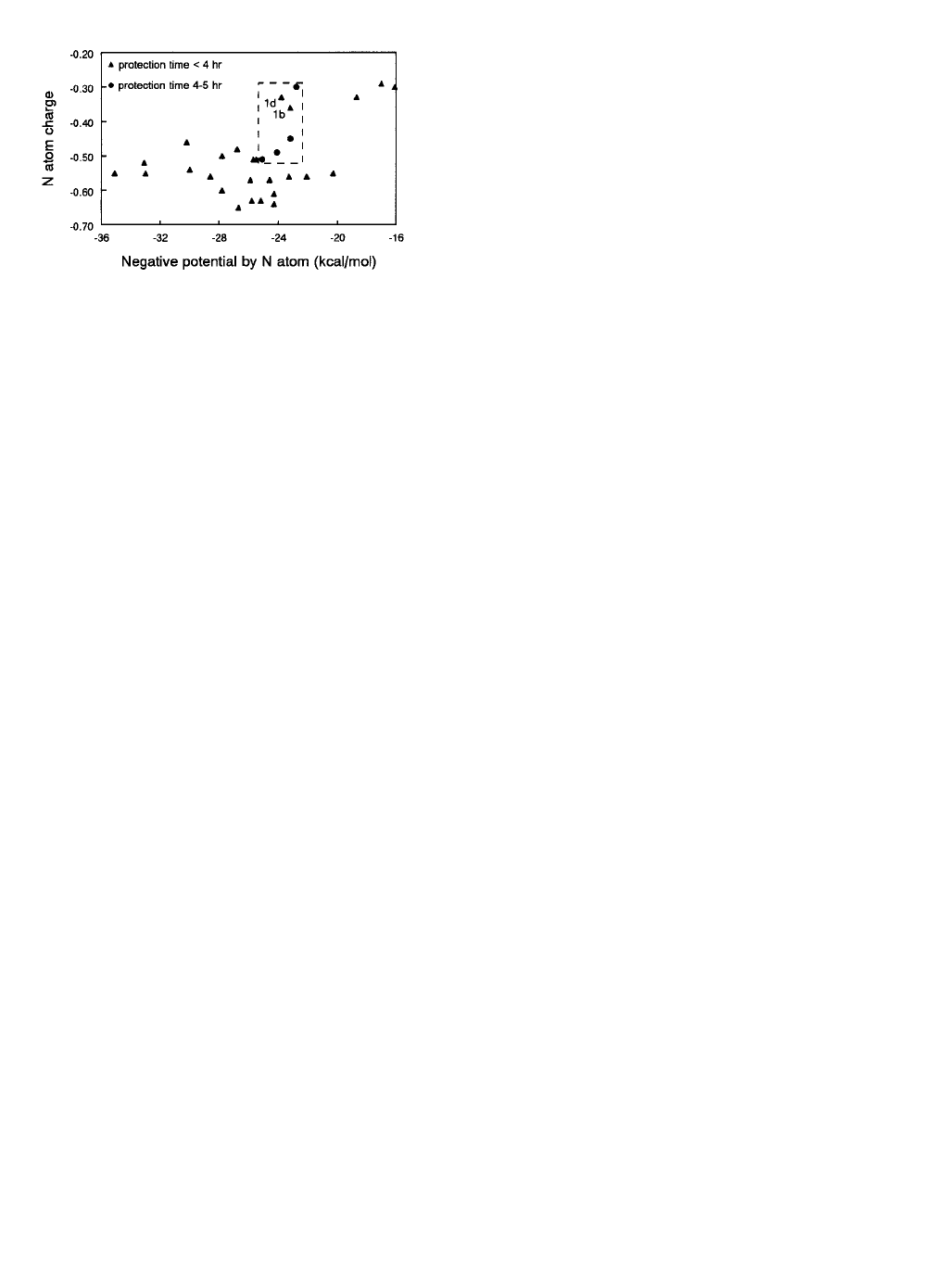
5
MOSQUITO REPELLENT POTENCY
F
IGURE
2. Plot of the atomic charge of the amide nitrogen atom
versus the maximum negative potential by the amide nitrogen atom,
showing that all compounds that protect less than 4 hr, except for
compounds 1b and 1d, have values that fall outside the range of
values of the compounds that protect for at least 4 hr (see box with
dashed lines).
though the values of the amide nitrogen atom for compounds
1b and 1d fall within the numerical ranges for high efficacy,
their dipole moment (compound 1d) or their electrostatic po-
tential by the amide oxygen atom (compound 1b) fall outside
these optimal ranges. Thus, this group of electronic proper-
ties serves as discriminators of efficacy of repellency.
Interestingly, when the values of the calculated electronic
properties are outside of the numerical ranges of the most
potent compounds, they are usually too low for the maxi-
mum negative potential that is located by the amide oxygen
atom, too low for the atomic charge of the amide oxygen
atom, too high for the atomic charge of the carbonyl carbon
atom, and too low for the atomic charge of the amide nitro-
gen atom.
The test data of Suryananarayana and others
23
show that
DEET analogs with a secondary amine exhibit only short
protection times. This was reflected in high maximum pos-
itive electrostatic potentials on the surface of the molecule
and a highly negative atomic charge at the nitrogen atom.
The biologic data used in this study were limited to the
yellow fever mosquito Ae. aegypti; therefore, the results may
be valid for only this arthropod. The rank order of effec-
tiveness of DEET versus other repellent compounds has
been shown to be similar for Ae. aegypti and the malaria
parasite Anopheles stephensi.
26–29
The electronic properties of the amide group (N-C
5
O at-
oms) seem to be the key in determining the duration of pro-
tection against mosquito bites. The substituents attached to
the carbon and nitrogen atoms of the amide group together
influence the electronic properties of the amide group. Thus,
a balance of polarity between the two parts of the molecule
is an important contributing factor for potent repellent activ-
ity. Our investigation shows that the numerical values of the
electronic properties of the amide group fall into discrete
ranges for a lengthy duration of protection. These optimal
values are now being used as a guide for the synthesis and
testing of new potential insect repellents.
Acknowledgments: We thank the National Research Council, Wash-
ington, DC for assistance in the support of Dr. Da Ma and Dr. Apur-
ba K. Bhattacharjee. We also thank Dr. Ralf Brueckner and Dr. Mark
Marino for assistance in the use of S-Plus.
Authors’ addresses: Da Ma and Raj K. Gupta, Department of En-
tomology, Division of Communicable Diseases and Immunology,
Walter Reed Army Institute of Research, Washington, DC 20307-
5100. Apurba K. Bhattacharjee and Jean M. Karle, Department of
Pharmacology, Division of Experimental Therapeutics, Walter Reed
Army Institute of Research, Washington, DC 20307-5100.
Reprint requests: Jean M. Karle, Department of Pharmacology, Di-
vision of Experimental Therapeutics, Walter Reed Army Institute of
Research, Washington, DC 20307-5100.
REFERENCES
1. Gupta RK, Rutledge LC, 1994. Role of repellents in vector
control and disease prevention. Am J Trop Med Hyg 50: 82–
86.
2. McCabe ET, Barthel WF, Gertler SI, Hall SA, 1954. Insect re-
pellents. III. N,N-diethylamides. J Org Chem 19: 493–498.
3. Gilbert IH, Gouck HK, Smith CN, 1955. New mosquito repel-
lents. J Econ Entomol 48: 741–743.
4. Skinner WA, Johnson HL, 1980. The design of insect repel-
lents. Arien EJ, ed. Drug Design. Volume X. New York: Ac-
ademic Press, 277–302.
5. Moody RP, Riedel D, Ritter L, Franklin CA, 1987. The effect
of DEET (N,N-diethyl-m-toluamide) on dermal persistence
and absorption of the insecticide fenitrothion in rats and mon-
keys. J Toxicol Environ Health 22: 471–479.
6. Watanabe K, Shono Y, Kakimizu A, Okada A, Matsuo N, Satoh
A, Nishimura H, 1993. New mosquito repellent from Euca-
lyptus camaldulensis. J Agri Food Chem 41: 2164–2166.
7. Morton FA, Travis BV, Linduska JP, 1947. Results of screening
tests with materials evaluated as insecticides, miticides and
repellents at Orlando Laboratory, April, 1942 to April, 1947.
US Dept Agr Bur Entomol Plant Quaran: E-733.
8. Roadhouse LAO, 1953. Laboratory studies on insect repellency.
Can J Zool 31: 535–546.
9. Gouck HK, Hall SA, Smith CN, Gilbert IH, 1957. Repellency
of homologous series of cyclohexane aliphatic acids and am-
ides. J Econ Entomol 50: 175–177.
10. Garson LR, Winnike ME, 1968. Relationships between insect
repellency and chemical and physical parameters-a review. J
Med Entomol 5: 339–352.
11. Davis EE, Rebert CS, 1976. Lactic acid-sensitive receptor on
the antennae of mosquito. J Econ Entomol 105: 1058–1061.
12. Johnson HL, Skinner WA, Maibach HI, Pearson TR, 1967. Re-
pellent activity and physical properties of ring-substituted N,
N-diethylbenzamide. J Econ Entomol 60: 173–176.
13. Rayner HB, Wright RH, 1966. Far infrared spectra of mosquito
repellents. Can J Entomol 98: 76–80.
14. Johnson HL, Skinner WA, Skidmore D, Maibach HI, 1968.
Topical mosquito repellents. II. Repellent potency and dura-
tion in ring-substituted N,N-dialkyl- and aminoalkylbenza-
mides. J Med Chem 11: 1265–1268.
15. Dethier VG, 1956. Repellents. Annu Rev Entomol 1: 181–202.
16. Christophers SR, 1947. Mosquito repellents being a report of
the work of the mosquito repellent inquiry. J Hyg 45: 176–
231.
17. Bunker CWO, Hirschfelder AD, 1925. Mosquito repellents. Am
J Trop Med 5: 359–383.
18. Piper DE, Hall RH, Wright GF, 1951. Chemistry of insect re-
pellency. Chem Can 3: 97–98.
19. Dethier VG, 1947. Chemical Insect Attractants and Repellents.
Philadelphia: Blakiston Co.
20. Sugawara R, Tominaga Y, Suzuki T, 1977. Effects of ring un-
saturation on the activity of propyl cyclohexaneacetate as an
attractant for the German cockroach. Insect Biochem 7: 483–
485.
21. Alexander BH, Beroza M, 1963. Aliphatic amides of cyclic
amines and tolyl maleimides as mosquito repellents. J Econ
Entomol 56: 58–60.
22. McIver SB, 1981. A model for the mechanism of action of the
repellent DEET on Aedes Aegypti (Diptera: Culicidae). J Med
Chem 11: 357–361.
23. Suryanarayana MVS, Pandey KS, Parkash S, Raghuveeran CD,