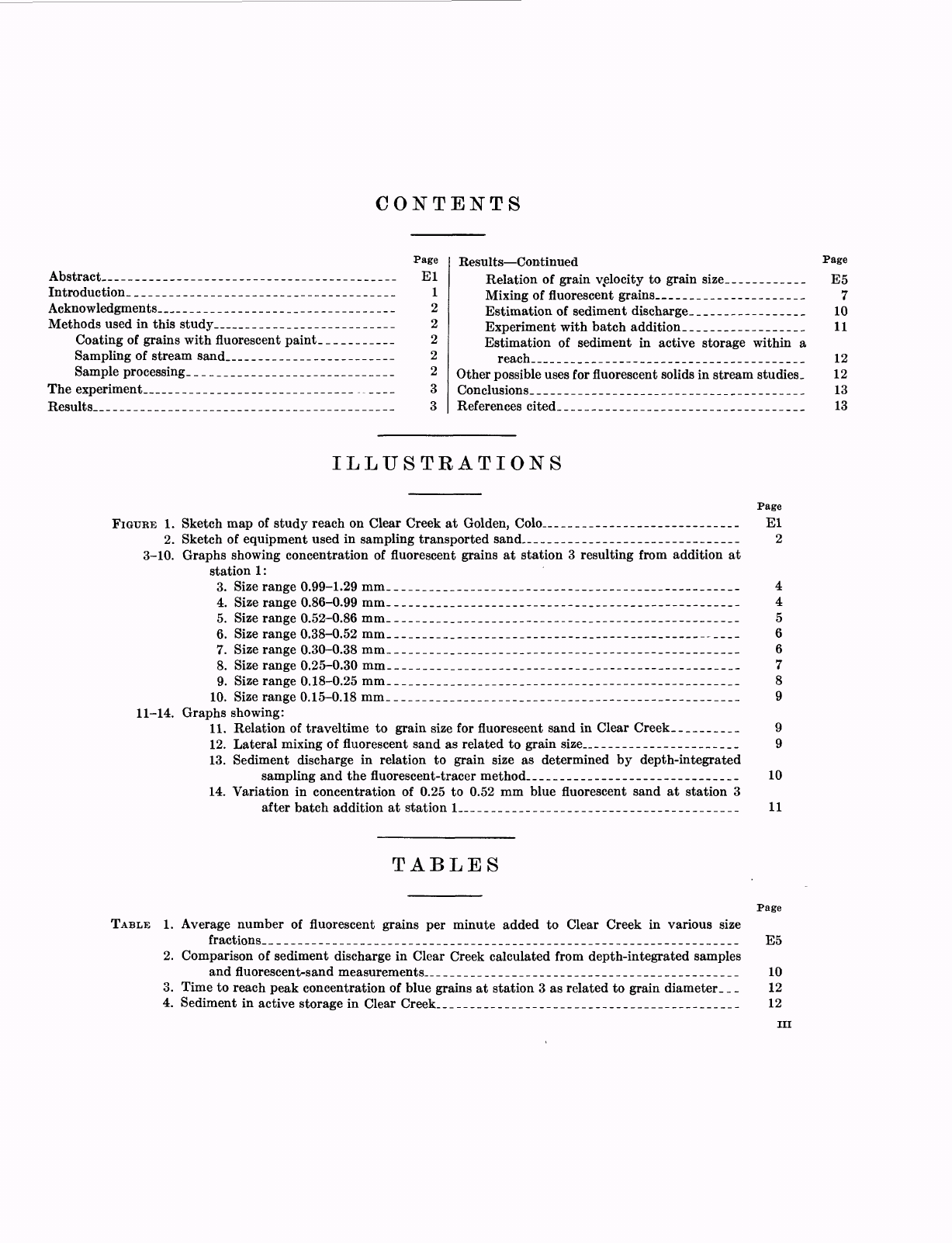
CONTENTS
Abstract-.-
-_---___-------------------_-
Introduction.
___________________________
Acknowledgments__
____________________
Methods
used
in
this
study_____________
Coating
of
grains
with
fluorescent
paint.
Sampling
of
stream
sand____________
Sample
processing.__________________
The
experiment_______________________
Results_-_-______---__-____--_-----___
Page
El
1
2
2
2
2
2
3
3
Results Continued
Relation
of
grain
velocity
to
grain
size___-_--____-
E5
Mixing
of
fluorescent
grains.-____________________
7
Estimation
of
sediment
discharge.________________
10
Experiment
with
batch
addition__________________
11
Estimation
of
sediment
in
active
storage
within
a
reach______________________________________
12
Other
possible
uses
for
fluorescent
solids
in
stream
studies.
12
Conclusions._______________________________________
13
References
cited.___________________________________
13
ILLUSTRATIONS
Page
FIGURE
1.
Sketch
map
of
study
reach
on
Clear
Creek
at
Golden,
Colo_._______--_____-_----____-_-
El
2.
Sketch
of
equipment
used
in
sampling
transported
sand______________________________
2
3-10.
Graphs
showing
concentration
of
fluorescent grains
at
station
3
resulting
from
addition
at
station
1:
3.
Size
range
0.99-1.29
mm-_-_-_____-_---_-___----_---__-__--_---_-----_--__-
4
4.
Size
range
0.86-0.99
mm-__-______-____-____-___-_-_____---____--_-----___-
4
5.
Size
range
0.52-0.86
mm_--_-_-_____-____-----_----_-_-_-------_-----_-_-_-
5
6.
Size
range
0.38-0.52
mm__-_________________-_-_-_-_-_-------_-_---________
6
7.
Size
range
0.30-0.38
mm__-_____________________---_-_--__-_-_-_--__-______
6
8.
Size
range
0.25-0.30
mm-___-_--_______--_-_---_-_-_-_---_-__----_-----___-
7
9.
Size
range
0.18-0.25
mm_______________________-__________-_--___-_________
8
10.
Size
range
0.15-0.18
mm._______________________________-------_-_-___-____
9
11-14.
Graphs
showing:
11.
Relation
of
traveltime to
grain
size
for
fluorescent
sand
in
Clear
Creek..________
9
12.
Lateral
mixing
of
fluorescent
sand
as
related
to
grain
size.._____________________
9
13.
Sediment
discharge
in
relation to
grain
size
as
determined
by
depth-integrated
sampling
and
the
fluorescent-tracer
method__________-_-__-_---_-___-____.
10
14.
Variation
in
concentration
of
0.25
to
0.52
mm
blue
fluorescent
sand
at
station
3
after
batch
addition
at
station
1_______________________________________
11
TABLES
TABLE
1.
Average
number
of
fluorescent
grains
per
minute
added to
Clear
Creek
in
various
size
fractions.-______________________________________________________---_-______
2.
Comparison
of
sediment
discharge
in
Clear
Creek
calculated
from
depth-integrated
samples
and
fluorescent-sand
measurements
____________________________________________
3.
Time
to
reach
peak
concentration
of
blue
grains
at
station
3
as
related
to
grain
diameter.
_ _
4.
Sediment
in
active
storage
in
Clear
Creek.___________________________________________
Page
E5
10
12
12