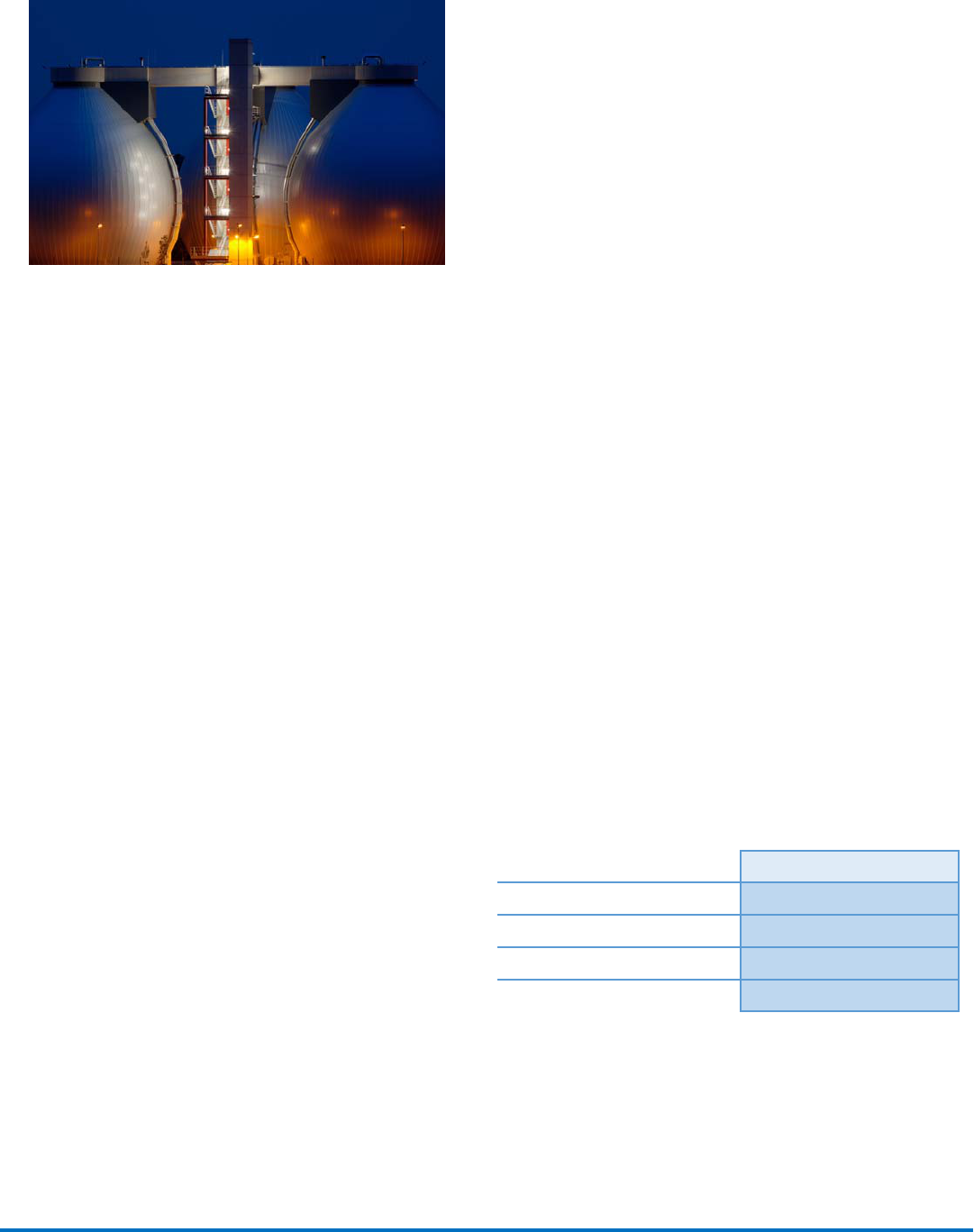
Higher-value fuels such as compressed natural gas (CNG),
liquid natural gas (LNG), or methanol may also be produced
from biogas. While these fuels are considered higher-value,
the processes for producing and using CNG, LNG, and
methanol are more complex in comparison to using biogas
for heat and power generation. As technology advances,
the use of biogas to produce these higher-value fuels may
become more common.
Prior to use, biogas must be pretreated to remove impurities
and other substances which may cause maintenance and
process efficiency issues. The water vapor present in biogas
reduces process effectiveness, and may be removed by
sloping biogas piping toward sediment/drip traps that
collect condensed water vapor for disposal.
Hydrogen sulfide, when combined with water vapor, forms a
weak acid that may damage biogas piping or equipment.
Passing biogas through wood chips impregnated with iron
sponge, biological scrubbers, or activated carbon help
remove hydrogen sulfide from biogas. Liquid phase
oxidation is an alternative removal method.
Siloxanes present in biogas convert to silicon dioxide
particles or sand when heated (for example, in a boiler,
engine, or turbine). These particles may cause damage and
reduce equipment life. Adsorption using activated carbon
or condensation may be employed to remove siloxanes.
Beside biogas, digestion also produces biosolids. Biosolids
may be conveyed to a secondary digester (if present) or
the mechanical dewatering process. Alternatively, — and
depending on regulations and WRRF operation — the
biosolids may be collected and hauled to an offsite location
for final disposal or further processing.
Secondary digester tanks may be employed to enable
liquid–solid separation of biosolids. This separation produces
a liquid called supernatant as well as thickened biosolids.
Supernatant is returned to the head of the WRRF (usually the
headworks), while the thickened biosolids may be
processed further or hauled offsite for final disposal.
Dewatering is the most common form of biosolids processing
post-digestion. The objective of dewatering is to reduce the
water content of biosolids; this reduces hauling costs. The
dewatered “cake” that is generated from dewatering may
be landfilled or, depending on the quality of the cake,
added to soil as an amendment. Numerous technologies
are available to mechanically dewater solids; common
equipment types include centrifuges, screw presses, belt
filter presses, and rotary presses. Solids drying beds are a
more passive dewatering approach and require more
space and additional labor to produce dewatered cake.
The liquid removed from solids during dewatering typically is
returned to the head of the WRRF.
Incineration is an alternative form of biosolids processing
and may be accomplished using multiple hearth reactors or
fluidized bed reactors. End products include carbon dioxide,
water, and ash. The objectives of incineration include
volume reduction in preparation for final disposal and
energy recovery.
DESIGN
There are three main stages of anaerobic digestion:
hydrolysis, fermentation, and methanogensis. All three occur
simultaneously and in the same vessel.
During hydrolysis, cells that were instrumental in biological
treatment in the liquid train are broken down into a soluble
form. Fermentation follows hydrolysis. During this stage, the
soluble products formed during hydrolysis are converted to
a mixture of volatile fatty acids (a process called
acidogensis) and, then, the mixture of volatile fatty acids is
converted primarily to acetic acid, carbon dioxide, and
hydrogen (a process called acetogenisis). The final stage is
methanogensis, which involves the conversion of acids and
hydrogen (formed during fermentation) to methane and
carbon dioxide. The proper design of anaerobic digesters
helps maximize the effect and success of each of these
three stages.
While there are numerous styles and operational strategies,
most anaerobic digesters are operated as single stage
mesophilic reactors. Table 2 (p. 3) provides typical design
criteria for high rate mesophilic digesters.
Table 2. Typical high rate mesophilic digester design criteria
Numerous factors greatly affect the construction and
operation of anaerobic digesters and must be considered
during design. The following section summarizes a few of
these factors.
Copyright © 2017 Water Environment Federation. All Rights Reserved. 3
WSEC-2017-FS-002—Municipal Resource Recovery Design Committee—Anaerobic Digestion Fundamentals
Table adapted from Design of Municipal Wastewater Treatment Plants
(Manual of Practice No. 8), published by the Water Environment
Parameter
Typical target range
Temperature
95°F to102°F
Volatile solids loading rate
0.12 to 0.16 lb/ft
3
•day
Feed percent solids
4% to 7%
Solids retention time
15 to 20 days
WEF Photos